Slot Joker123 Gaming
Couldn't load pickup availability

Selamat datang di situs Joker123 slot yang merupakan penyedia sajian game slot paling populer pada masa sekarang ini. Hal ini tentu saja dikarenakan, layanan dari slot joker123 gaming memberikan sebuah akses untuk pemain dalam melakukan daftar. Maupun login joker123 apk melalui smartphone. Para slotmania juga bisa merasakan keseruan bermain slot online uang asli dari setiap game slot yang sudah disediakan oleh provider slot joker123 gaming. Seperti yang kita ketahui, kalau saat ini perjudian slot sangatlah gemar, dan banyak dilakukan oleh setiap penjudi di seluruh penjuru dunia. Terutama di Indonesia. Dimana, hal ini terjadi karena permainan slot sendiri bisa dimainkan dengan menggunakan modal yang kecil. Namun untuk tawaran hadiah yang disediakan tidaklah main-main. Dimana penyedia judi slot terpercaya seperti joker gaming saat ini telah menyediakan jackpot slot sebesar miliaran rupiah. Hal ini tentu saja menjadi sebuah keseruan dalam bermain slot online uang asli menggunakan provider dari slot joker123 gaming.
Untuk soal keamanan, Tentu tidak perlu lagi dipertanyakan, dimana layanan dari slot joker123 gaming kini telah dilengkapi dengan berbagai izin resmi dari badan keamanan perjudian dunia slot online. Seperti, MGA, PAGCOR, dan Gambling Commission. Setiap sajian game yang ditawarkan oleh joker123 juga sangatlah bervariasi, dimana pemain bisa merasakan permainan slot dengan mudah dimana saja, dan kapan saja diinginkan. Karena setiap permainan yang disediakan oleh joker123 gaming akan aktif selama 24 jam. Jadi untuk kalian para pecinta judi slot bisa menikmati berbagai permainan yang tersedia kapan jam.
Joker123 Gaming Menyediakan Game Slot Gacor Hari Gampang Menang
Joker123 Gaming selaku perusahaan perjudian slot online yang sudah ada sejak lama. Tentu saja selalu menyediakan permainan slot gacor hari ini. Dimana, setiap game yang disajikan bisa dengan mudahnya untuk dimenangkan. Karena game yang disediakan oleh joker123 slot memiliki sebuah persentase kemenangan yang sangat tinggi dibandingkan dengan berbagai provider lainnya. Hal ini bisa kita lihat sendiri dari RTP Live rata-rata dari setiap permainan yang disediakan oleh joker123 gaming. Semua mesin slot joker123 juga memberikan jaminan kepada setiap member, bahwa setiap permainan joker123 slot gacor hari ini dijamin anti rungkat. Itulah kenapa sampai sekarang ini, banyak dari para pemain yang lebih suka dengan menggunakan situs joker123 slot dalam menikmati berbagai sajian gaming uang asli yang benar-benar bisa mendatangkan cuan kepada setiap sloter.
Joker123 gaming juga menjadi andalan bagi setiap pemain di asia. Bagaimana tidak? Hal ini terjadi karena cara bermain, dan juga fitur yang disediakan sangatlah lengkap. Dimana, para pemain bisa mencoba fitur gratis berupa sajian game slot demo gratis no deposit. Untuk memainkan berbagai game slot joker123 yang sudah disediakan. Dan untuk mencoba fitur dari demo slot joker123 gaming juga sangatlah mudah. Dimana, para pemain bisa langsung menggunakan situs kami, dan langsung memilih menu demo joker123 gaming. Sajian game joker123 juga sangatlah lengkap, dan pastinya bisa dimainkan melalui berbagai perangkat. Tanpa harus melakukan pembukaan akun demo slot dahulu. Itulah, kenapa saat ini joker123 gaming slot selalu menjadi sebuah penyedia judi slot online terbaik di Indonesia.
Login Joker123 Bisa Langsung Diakses Lewat Apk Smartphone
Login Joker123 saat ini menyediakan sebuah fasilitas kepada slotmania. Berupa apk smartphone yang bisa langsung di download, dan dimainkan tanpa deposit. Dimana, joker123 slot selaku situs judi slot online terbesar di asia. Tentu saja banyak memberikan fitur, serta kemudahan kepada pemain dalam menikmati sajian game yang disediakan. Tidak hanya itu saja, joker123 apk juga menjadi sebuah permainan resmi yang kini banyak disediakan oleh setiap agen slot online di Indonesia. Sehingga akan memudahkan pemain untuk menikmati berbagai permainan slot joker123 dengan mudah lewat situs judi online yang kini sudah banyak tersedia di internet.
Untuk melakukan login joker123 dan memainkan permainan slot online uang asli. Setiap pemain haruslah melakukan pendaftaran akun, dan juga menyediakan berbagai data untuk melakukan pembelian chips dengan cara transfer menggunakan bank lokal di Indonesia. Atau bisa juga menggunakan berbagai E-Wallet yang sah oleh bank indonesia. Seperti, DANA GOPAY, LINK AJA, atau bisa menggunakan Pulsa Telkomsel tanpa rate.
Untuk melakukan pembukaan akun slot joker123 gaming. Kalian bisa mengikuti tata cara dibawah ini.
- Masuk Ke Situs joker123
- Isi Formulir Pendaftaran
- Masukkan User ID, Password, Nama Rekening, Nomor Rekening, dan Nomor WA
- Jika Semua Kolom Pendaftaran Sudah Terisi, Maka Pastikan Kembali, Kalau Semua Data Yang Diisi Sudah Benar
- Klik Tombol Daftar
Jika sudah selesai melakukan pendaftaran akun joker123 slot. Maka anda wajib untuk melakukan deposit awal dengan pilihan metode setor dana yang sudah tersedia. Jika sudah melakukan transfer ke rekening situs joker123 slot. Maka langkah yang harus anda lakukan adalah melakukan pengisian formulir deposit. Lalu anda bisa menikmati berbagai sajian game slot joker123 gaming yang sudah disediakan. Setiap permainan yang sudah ada list joker gaming. Bisa anda mainkan dengan modal sebesar Rp. 10.000 saja.
Keuntungan Bermain Slot Joker Gaming 123
Selain mudah untuk melakukan daftar joker gaming 123. Tentu saja para pemain juga akan diberikan banyak sekali keuntungan dalam bermain slot joker123. Dimana hal ini dilakukan untuk memuaskan pemain dalam menikmati game slot yang sudah disediakan. Dan pastinya setiap tawaran permainan joker gaming 123 yang tersedia pastinya bisa anda nikmati, tanpa syarat apapun itu.
- Tersedia Joker123 Apk Smartphone
- Download Joker123 Dimana Saja
- Fasilitas Slot Joker123 Deposit Pulsa
- Fitur Demo Slot Garis No Deposit
- Deposit & Withdraw Super Cepat
- Terjamin Fairplay
- Bantuan Customer Service Live Chat 24 Jam
Nah itulah keuntungan dalam melakukan daftar joker123 slot dan memainkan permainan yang sudah disajikan. Setiap permainan yang sudah ada pastinya bisa anda nikmati dengan seru, bersama teman, maupun keluarga kapan saja diinginkan.
4 Jenis Permainan Joker123 Gaming Terbaru Mudah Maxwin
Joker123 selaku situs slot online terbesar dan terkemuka di dunia. Pastinya menyediakan sajian game paling gacor dari joker123 gaming. Hal ini tentu saja menjadi salah satu alasan kenapa sampai sekarang ini, banyak dari para penjudi slot yang lebih memilih untuk memainkan joker123 gaming di situs joker123. Berikut inilah sajian game slot gacor dari joker gaming.
Joker123 NEPTUNE TREASURE
Slot joker123 neptune trasure merupakan salah satu game slot bertemakan sebuah lautan yang memiliki raja, berupa poseidon. Slot joker ini menjadi salah dari satu permainan populer, dan saat ini banyak dimainkan oleh para pemain. Hal ini tentu saja dikarenakan oleh berbagai alasan. Salah satunya adalah tawaran jackpot besar yang disediakan. Dimana setiap pemain bisa menikmati sajian game dengan minimal spin sebesar 200 rupiah. Dan berkemungkinan besar meraih mega jackpot sebesar 200 juta rupiah. Tentu saja game slot joker gaming satu ini menjadi satu-satu game yang berani memberikan jackpot terbesar dari para pesaing lainnya. Itulah kenapa sampai sekarang ini kenapa joker123 gaming menjadi provider ternama dengan jackpot terbesar yang disediakan.
RTP Live: 90%
Fitur Slot: Progressive Jackpot
Rilis: 29 Oktober 2021
Minimal Spin: 200 rupiah
Maksimal Jackpot: 200 Juta Rupiah
Joker123 CRYPTOMANIA
Game slot cryptomania pertama kali dirilis pada tahun 2020 lalu, menjadi salah satu permainan terbaik dengan latar tema tambang koin. Dimana, game slot joker123 menyajikan permainan dengan persentase kemenangan besar. Selain itu setiap sloter juga bisa memanfaatkan berbagai fitur dari mesin slot yang satu ini. Yaitu berupa scater yang dapat dibeli dengan harga terjangkau. Itulah kenapa sampai sekarang ini, banyak dari pemain yang menggunakan permainan joker123 cryptomania sebagai akses bermain dengan kemenangan yang mudah untuk didapatkan.
RTP Live: 98%
Fitur Slot: Scatter
Rilis: 5 Mei 2020
Minimal Spin: 200 rupiah
Maksimal Jackpot: 150 Juta Rupiah
Joker123 CAISEN RICHES
Caisen riches yang merupakan sebuah game slot joker gaming 123 menjadi andalan para penjudi slot di dunia. Bagaimana tidak? Dengan menyediakan permainan slot online uang asli. Kini setiap pemain bisa merasakan keseruan dalam menikmati permainan yang disediakan. Caisen riches sendiri menghadirkan tema berupa dewa hoki dari china yang pastinya, untuk setiap slotmania yang menikmati permainan ini akan diberikan keberuntungan beruntun dari setiap jackpot yang disediakan. Dan sampai sekarang ini game slot Caisen riches menjadi salah satu permainan populer di asia. Terutama di Indonesia dan Thailand.
RTP Live: 97%
Fitur Slot: Mega Jackpot
Rilis: 1 Agustus 2019
Minimal Spin: 200 rupiah
Maksimal Jackpot: 180 Juta Rupiah
Joker123 MAJAPAHIT
Slot majapahit mengadopsi tema berupa kerajaan yang berasal dari Indonesia. Dimana, slot joker123 sendiri memberikan nilai sempurna pada mesin slot yang satu ini. Bagaimana tidak? Slot majapahit sendiri memiliki persentase kemenangan yang sangatlah besar. Dan untuk para pemain yang menikmati permainan ini sudah pastinya akan jauh lebih mudah untuk mendapatkan mega jackpot yang sudah disediakan oleh joker gaming 123. Tidak hanya itu saja, setiap pemain juga bisa merasakan kemudahan, serta keseruan dalam menikmati sajian game yang disediakan. Dan untuk mencoba memainkan demo slot ini. Setiap sloter bisa langsung melakukan daftar joker123 menggunakan layanan joker123.
RTP Live: 98.95%
Fitur Slot: Scatter
Rilis: 18 Agustus 2018
Minimal Spin: 200 rupiah
Maksimal Jackpot: 300 Juta Rupiah
Share
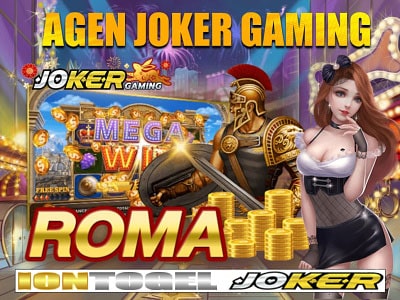